Binary Neutron Star Mergers as the Production Site of Gold, Platinum, and Rare Earth Elements
| Science
Researchers at NAOJ and the University of Tokyo revealed that gold, platinum, and rare earth elements are likely produced and distributed through binary neutron star mergers. These elements are examples of a group known as r-process elements, which are produced by rapid neutron capture reactions. It has been a mystery where in nature the extreme conditions needed for this process could occur. The research team determined that r-process elements ejected from neutron star mergers at very high speeds (10 – 30 % the speed of light) quickly pervade their entire host galaxy, and can account for the chemical signature observed in stars in the Milky Way Galaxy and nearby dwarf galaxies.
In addition to elucidating the origins of valuable metals, this research can be used to estimate the rate of neutron star mergers from the signature they leave in the chemical composition of stars. Since neutron star mergers are believed to emit gravitational waves, this rate is an important factor for the success of advanced gravitational wave detectors like KAGRA, which is currently under construction. This research indicates that when it is fully operational in the 2020s, KAGRA can be expected to detect signals from neutron star mergers about once every one or two months through international collaboration with other advanced detectors such as LIGO and VIRGO.
These results were published in a European peer review journal “Astronomy and Astrophysics”.
BACKGROUND
A few minutes after the beginning of the Universe, the only primordial elements available were hydrogen, helium, and a little lithium. Heavier elements (with larger atomic numbers) such as carbon were synthesized later in the hot, dense regions inside stars. This research dealt with elements heavier than iron (Fe) which are synthesized through repeated neutron capture reactions with seed elements (like iron or lighter elements). Among these heavy elements, the ones requiring the highest neutron densities are called r-process elements. R-process elements include gold, platinum, and rare earth elements. Until now, astronomers had been unable to determine where the conditions to produce these r-process elements occurred.
There are two likely candidates for the production site. One is a type of supernova explosion initiated by the collapse of the central cores of massive stars (stars with greater than 10 times the mass of the Sun), which eventually form neutron stars at the center. But recent theoretical studies have shown that matter ejected from neutron stars does not contain enough neutrons to synthesize r-process elements.
The other candidate is neutron star mergers. As binary neutron stars circle around each other, they lose energy through gravitational waves and slowly fall towards one another, taking at least 10 million years to finally collide and merge into a single object. Results of recent numerical simulations have shown that this merger event ejects a large amount of r-process elements. For example, one merger event ejects an amount of gold equal to 70 times the entire mass of the Earth. Actually, a short duration gamma-ray burst (GRB 130603B) detected in 2013 is thought to be the result of such a neutron star merger. Observations in visible and near infrared light confirmed that r-process element ejection was associated with this event.
Nevertheless, this second hypothesis had a problem. Since a neutron star merger ejects exclusively r-process elements, next generation stars formed from gas influenced by this event are expected to contain an extremely large amount of r-process elements relative to other heavy elements. But, we have not found any such r-process enriched stars anywhere in the Universe.
THIS STUDY
The researchers focused their attention on the abundance of elements in nearby dwarf galaxies. In this study, iron content was measured to trace time, because the iron content in a galaxy continuously increases due to numerous supernovae. In contrast, while rare a single neutron star merger produces a sharp increase in the r-process element content. Because the total number of stars in the dwarf galaxies is much smaller than the number found in the Milky Way, these dwarf galaxies have probably experienced few or no neutron star mergers. Thus it is expected that the chemical signature in the stars will show no increase in r-process elements, or will show sudden, discrete increases in the content of r-process elements as the iron content increases.
In recent years large 8-10 m class telescopes, including the Subaru Telescope, have enabled us to measure the chemical compositions of individual stars in dwarf galaxies out to a distance of 800,000 light-years.
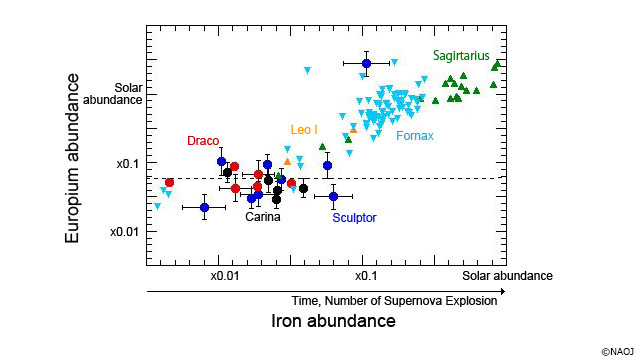
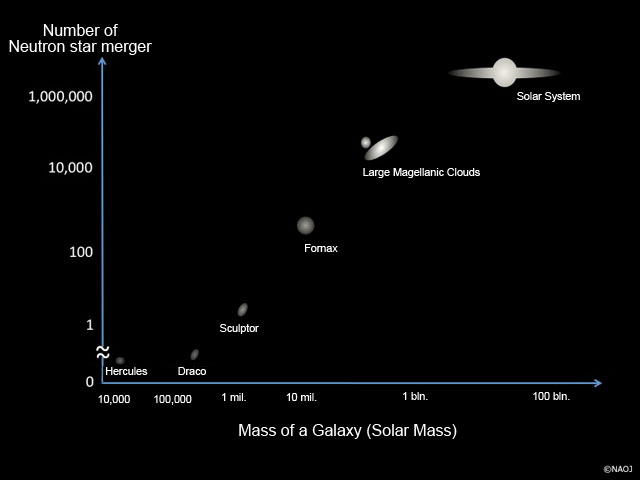
The team compared the amount of europium (Eu), a representative r-process element, on the surfaces of stars in some faint dwarf galaxies. They found that in galaxies with low levels of europium, the amount remains constant in all stars, even as the iron content of the stars increases. This means that there was a small amount of europium present when these dwarf galaxies formed and there has been no subsequent event producing additional r-process elements in these dwarf galaxies. At the same time, increasing Fe contents indicate there have been numerous supernova explosions in these galaxies. This suggests that supernovae do not produce r-process elements.
On the other hand, in relatively luminous (massive) dwarf galaxies, the amount of europium is enriched in younger stars. This indicates that these galaxies have experienced r-process production events over their histories. Furthermore, it was found that the event rate needed to reproduce the characteristics of europium found in the various galaxies is about 1/1,000 the expected supernova rate. This event rate is comparable to the estimated rate of neutron star mergers and thus supports neutron star mergers as the origin of r-process elements.
But the lack of extremely r-process enriched stars still provided a problem for the models. The researchers reconsidered how r-process elements would be ejected into interstellar space from a neutron star merger and found an error in previous assumptions.
Matter ejected from supernovae propagates through interstellar space for about 100 light-years as it mixes with the interstellar medium. On the galactic scale, this is a localized effect. Previous works have assumed the same mixing process for matter ejected from neutron star mergers. In truth, matter ejected from neutron star mergers can propagate further because of much higher velocities, i.e., about 10 to 30% of the speed of light. This matter is found to propagate distances more than 1000 times longer while zigzagging along tangled galactic magnetic fields. Because the r-process elements are spread over a wide area, the concentration is diluted and this prevents any one region from becoming too rich in r-process elements.
From calculations of the time evolution of r-process elements in the Milky Way Galaxy using this new propagation process model, we obtain results in good agreement with the observations. Now the hypothesis that neutron star mergers are the origin of r-process elements can be reconciled with all observational facts.
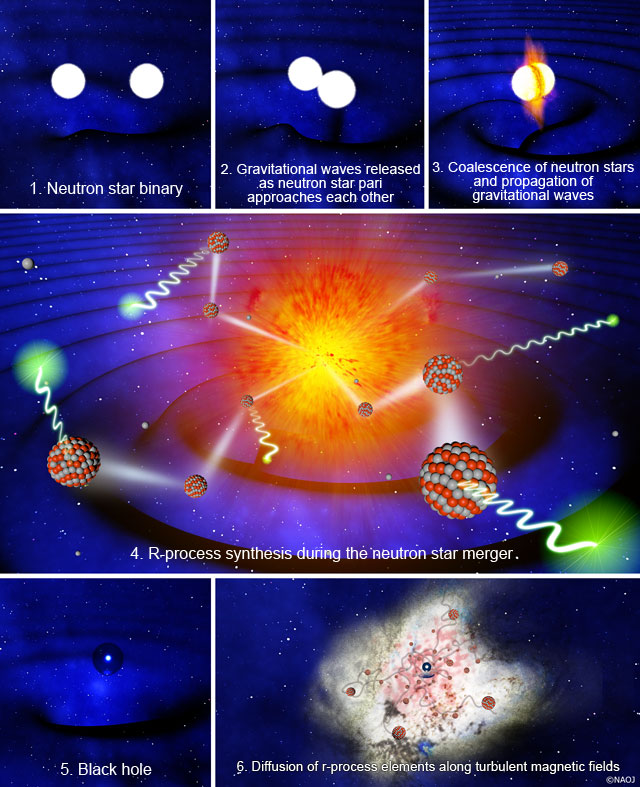
FUTURE PROSPECTS
It is expected that advanced detectors now under construction will detect gravitational waves from neutron star mergers. Thus it is important to have an accurate estimate of the event rate. According to the results of this study, the rate is estimated to be 10 to 20 events per million years in the Milky Way Galaxy. KAGRA will have the sensitivity to detect gravitational waves from a neutron star merger within a distance of 800 million light-years. Within this volume, there are about 1 million galaxies with sizes comparable to the Milky Way. So we can expect an event within KAGRA’s detection range every one or two months.
Though observational data of r-process elements in dwarf galaxies are essential to estimating the event rate of neutron star mergers, the data thus far obtained are still incomplete. We plan to perform observations to measure the tiny amount of r-process elements in individual stars in dwarf galaxies using the Subaru Telescope to build a more complete picture of this process.
Note
1. neutron star: an extremely compact star composed mainly of neutrons. The size is about 10 km and the mass ranges from 1.3 to 2 solar masses.
2.tangled magnetic fields: magnetic fields are ubiquitous in interstellar space. The gas there is turbulent and distorts the configuration of the magnetic fields.
3. gravitational wave: according to Einstein’s theory of General Relativity, space-time is distorted by the existence of energy and/or mass. Motion of the mass and/or asymmetrical changes in the energy generates gravitational waves, ripples in space-time which propagate at the speed of light.
Presenter
TSUJIMOTO, Takuji (JASMINE Project Office, NAOJ)
SHIGEYAMA, Toshikazu (Research Center for the Early Universe, the Graduate School of Science at the University of Tokyo)
Journal
Journal Title: Astronomy and Astrophysics
Article Title: Enrichment history of r-process elements shaped by a merger of neutron star pairs
Authors: Takuji Tsujimoto; Toshikazu Shigeyama
DOI Number: 10.151/0004-6361/201423751